Alkali-Silica Reaction (ASR) In Concrete
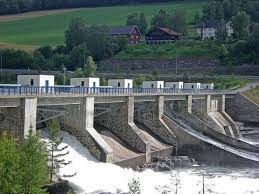
Introduction:
The alkali-silica reaction (ASR) is a complex chemical process that occurs in concrete and has significant implications for the durability and performance of concrete structures. ASR arises when alkalis present in cement react with reactive forms of silica in aggregates, resulting in the formation of a gel-like substance. This gel absorbs water, leading to the expansion and cracking of the concrete over time. Understanding and addressing ASR are crucial for engineers and practitioners in the field of concrete technology to ensure the long-term integrity and functionality of concrete structures.
ASR is an important topic of study due to its potential detrimental effects on concrete. The expansion and cracking caused by ASR can compromise the structural integrity of the concrete, resulting in reduced load-bearing capacity and safety risks. Additionally, the presence of cracks allows the ingress of harmful substances, such as water, chlorides, and sulfates, further accelerating the deterioration processes. This not only affects the aesthetics and functionality of the structure but also increases maintenance and repair costs.
By gaining a deep understanding of the causes and effects of ASR, engineers can implement preventive measures to minimize its occurrence. Factors such as the selection of suitable aggregates with low reactivity, control of alkali content in cement and admixtures, and optimization of the water-cement ratio can help mitigate the risk of ASR. Proper concrete mix design, curing methods, and ongoing monitoring are essential to prevent and manage ASR in concrete structures.
Addressing ASR is particularly crucial in regions with specific environmental conditions that enhance the reaction. Factors such as high temperatures, high humidity, and the presence of external sources of alkalis (such as de-icing salts or alkaline groundwater) can accelerate the ASR process. Understanding the influence of these environmental factors allows engineers to adapt concrete mix designs and construction practices accordingly.
Furthermore, ongoing research and advancements in concrete technology are continuously improving our understanding of ASR and its prevention. Researchers are exploring alternative cementitious materials, supplementary cementitious materials, and admixtures to control ASR and enhance the durability of concrete structures. The development of standardized testing methods and guidelines for ASR prevention facilitates the implementation of best practices and ensures consistency in addressing this issue.
In conclusion, understanding and addressing ASR is crucial for engineers and practitioners in the field of concrete technology. By comprehending the causes, effects, and preventive measures of ASR, the risk of structural damage, reduced service life, and increased maintenance costs can be minimized. Ongoing research and advancements in concrete technology further contribute to the development of effective strategies for ASR prevention. By prioritizing ASR mitigation, engineers can ensure the longevity, safety, and sustainability of concrete structures in the built environment.
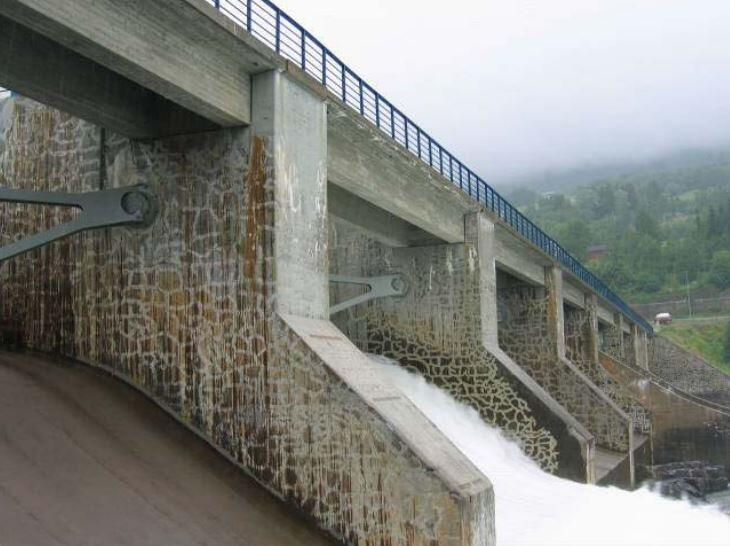
Chemical Structure and Reaction Mechanism:
Understanding the chemical structure and reaction mechanism of the alkali-silica reaction (ASR) is crucial for comprehending its impact on concrete and developing effective preventive measures. The reaction involves the interaction between alkali compounds in cement and reactive forms of silica present in aggregates.
Silica, in the form of silicon dioxide (SiO2), is a primary component of aggregates used in concrete. It exists in different forms, including non-reactive or crystalline silica and reactive or amorphous silica. Crystalline silica is chemically stable and does not participate in the ASR process. However, amorphous or reactive silica has a higher surface area and reactivity, making it prone to undergoing the ASR reaction.
Alkali compounds, such as sodium oxide (Na2O) and potassium oxide (K2O), are present in cement as a result of the manufacturing process. These alkalis can exist in various forms, including sodium hydroxide (NaOH) and potassium hydroxide (KOH), which readily dissociate in water to form highly alkaline solutions.
The chemical equation representing the alkali-silica reaction is:
3Na2O·2SiO2 + H2O → Na2O·2SiO2·3H2O (sodium-silicate gel)
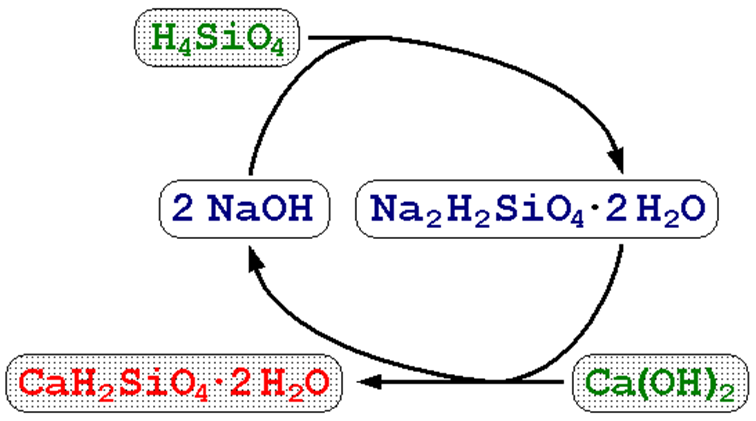
In this reaction, the alkalis from cement react with the reactive forms of silica in the aggregates in the presence of water. The alkalis disperse and dissolve in the water, creating a highly alkaline environment within the concrete. The dissolved alkalis then come into contact with the reactive silica, resulting in the formation of an alkali-silica gel.
The alkali-silica gel is a hydrated form of sodium silicate and has a gel-like structure. It possesses an expansive nature, meaning it can absorb water and swell over time. This swelling creates internal pressure within the concrete, leading to the expansion of the material and subsequent cracking. The cracks typically exhibit a map-like or spider-web pattern, propagating through the affected concrete.
The expansion and cracking caused by the ASR process create pathways for the ingress of moisture, aggressive chemicals, and other deleterious substances into the concrete. This can further accelerate deterioration processes, such as corrosion of reinforcing steel, chemical reactions, and loss of structural integrity.

Understanding the mechanism of ASR helps in developing preventive measures to mitigate its effects. By controlling the alkali content in cement, selecting non-reactive or low-reactive aggregates, and optimizing the water-cement ratio, the risk of ASR can be minimized. Additionally, the use of supplementary cementitious materials, such as fly ash or slag, can help mitigate the reactivity of silica in aggregates and reduce the potential for ASR.
In conclusion, the alkali-silica reaction involves the interaction between alkalis in cement and reactive forms of silica in aggregates. The formation of an alkali-silica gel and subsequent swelling and cracking of concrete characterize this reaction. Understanding the chemical structure and reaction mechanism of ASR is essential for implementing preventive measures and minimizing the risk of structural damage and durability issues in concrete structures.
Factors:
Understanding the factors that influence the alkali-silica reaction (ASR) is crucial for effectively managing and mitigating its effects on concrete structures. Several factors, including the components in the concrete mixture and environmental conditions, play significant roles in the occurrence and severity of ASR.
3.1 Influence of components in the concrete mixture:
The components within the concrete mixture can have a substantial impact on the development and extent of ASR. The type and characteristics of aggregates used in concrete are particularly important. Aggregates containing reactive forms of silica, such as opal, chert, or strained quartz, are more prone to ASR. It is essential to assess the reactivity of aggregates through standardized testing methods and consider their potential contribution to ASR when selecting and proportioning the concrete mix.
The cement composition also influences the potential for ASR. The alkali content in cement, especially sodium and potassium, can significantly contribute to ASR. Higher alkali levels in cement increase the availability of alkalis for reaction with reactive forms of silica. Therefore, controlling the alkali content in cement is vital in minimizing the risk of ASR.
The water-cement ratio is another important factor. Higher water-cement ratios provide more water for the alkali-silica reaction to occur, potentially accelerating the progression of ASR. Optimizing the water-cement ratio within suitable limits is essential for reducing the potential for ASR development.
The inclusion of supplementary cementitious materials (SCMs), such as fly ash or slag, can also influence ASR. SCMs can help mitigate ASR by reducing the availability of reactive forms of silica and alkalis for the reaction. The pozzolanic or cementitious properties of SCMs contribute to the formation of additional stable hydration products, which can bind the alkalis and silica, reducing their reactivity.
3.2 Effect of alkali-containing admixtures:
Certain admixtures used in concrete production, such as water reducers or accelerators, may contain alkalis. The presence of these alkali-containing admixtures can increase the overall alkali content in concrete, thereby enhancing the potential for ASR. It is crucial to carefully select and monitor the alkali content of admixtures to ensure they do not contribute excessively to alkali levels in the concrete mixture.
3.3 Influence of environmental factors:
Environmental conditions can significantly impact the occurrence and progression of ASR. Elevated temperatures and moisture levels facilitate the chemical reaction between alkalis and reactive silica, leading to faster gel formation and subsequent expansion. High humidity provides the necessary moisture for the reaction, while increased temperatures accelerate the reaction kinetics.
In regions with cold climates, the use of de-icing salts, which are often alkaline, can introduce additional alkalis into the concrete. Alkaline groundwater can also serve as a source of alkalis, further enhancing the likelihood of ASR. Considering these external sources of alkalis and implementing appropriate preventive measures is crucial to manage the risk of ASR effectively.
In conclusion, the components within the concrete mixture, including aggregates, cement composition, water-cement ratio, and the presence of alkali-containing admixtures, along with environmental factors, significantly influence the occurrence and severity of ASR. Careful selection and proportioning of materials, controlling alkali content, optimizing water-cement ratio, and considering environmental conditions are essential for mitigating the risk of ASR and ensuring the durability of concrete structures.
Consequences of Alkali-Silica Reaction:
The alkali-silica reaction (ASR) can have profound consequences on the performance and durability of concrete structures. Understanding these consequences is crucial for assessing the impact of ASR and implementing appropriate measures to mitigate its effects.
4.1 Swelling and cracking issues in concrete:
ASR-induced expansion of concrete can result in significant swelling and cracking. The gel-like alkali-silica reaction product occupies more volume than the original reactants, leading to internal pressure within the concrete. This pressure causes the concrete to expand, resulting in the development of cracks. These cracks typically exhibit a map-like or spider-web pattern and can propagate through the concrete, compromising its aesthetics, functionality, and structural integrity. The presence of these cracks also provides pathways for the ingress of harmful substances, such as water, chlorides, and sulfates, which can further accelerate deterioration processes.

4.2 Impact on concrete durability:
ASR not only leads to visible cracking but also significantly affects the long-term durability of concrete. The cracks created by ASR allow the penetration of moisture, aggressive chemicals, and deleterious substances into the concrete matrix. This can result in various forms of deterioration, including corrosion of reinforcing steel, chemical reactions, and loss of bond between aggregates and cement paste. These degradation processes reduce the overall durability and service life of the concrete structure.
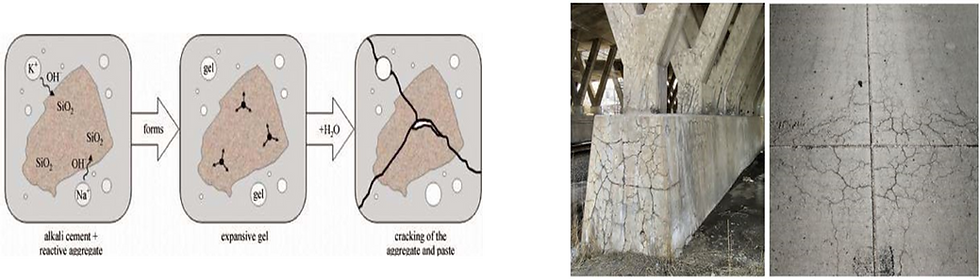
4.3 Structural damage and associated costs:
ASR-induced structural damage can have severe consequences for concrete structures. The compromised integrity of concrete elements jeopardizes the safety and performance of the structure. Depending on the severity of ASR, structural elements may require extensive repairs or even complete replacement. The financial implications of ASR are significant, encompassing not only the direct costs associated with repair materials, labor, and equipment but also the indirect costs related to disruptions, downtime, and potential legal liabilities.
Additionally, the costs associated with evaluating the extent of ASR damage and implementing preventive measures, such as monitoring programs or modifications to the construction practices, should be considered. The overall economic impact of ASR can be substantial, making it imperative to address this issue proactively through preventive measures and early detection to minimize the potential for structural damage and associated costs.
In conclusion, ASR can have severe consequences on concrete structures, including swelling, cracking, reduced durability, and structural damage. Understanding these consequences highlights the importance of implementing preventive measures, conducting regular inspections, and employing appropriate repair strategies to mitigate the detrimental effects of ASR. By addressing ASR early on, the long-term performance, safety, and durability of concrete structures can be safeguarded, ultimately reducing the financial burden associated with repairs and replacements.
Detection and Prevention:
Detecting and preventing alkali-silica reaction (ASR) is essential to ensure the durability and performance of concrete structures. By employing effective detection methods and implementing preventive measures, the risk and extent of ASR can be minimized.
5.1 Methods for detecting alkali-silica reaction:
Several methods can be utilized to detect the presence and extent of ASR in concrete structures. Visual inspections play a fundamental role in identifying signs of cracking, map-like patterns, or other indications of ASR. Petrographic analysis of concrete samples, involving microscopic examination of thin sections, can provide detailed information about the presence and distribution of reactive aggregates and ASR gel.
Non-destructive testing techniques can also aid in detecting ASR. Ultrasonic pulse velocity and electrical resistivity measurements can assess the internal condition of concrete and identify areas of potential ASR-induced damage. Accelerated laboratory tests, such as the concrete prism test or mortar bar test, can simulate the long-term effects of ASR in a short period, allowing for rapid assessment of concrete susceptibility to ASR.
5.2 Preventive measures:
Preventing ASR requires a comprehensive approach that addresses various aspects of concrete production and construction. Material selection is crucial, with particular attention given to the reactivity of aggregates. Non-reactive or low-reactivity aggregates should be preferred to minimize the risk of ASR. Additionally, controlling the alkali content in cement and admixtures is essential. By using low-alkali cements and carefully monitoring the alkali content of admixtures, the overall alkali load in the concrete mixture can be reduced, reducing the potential for ASR.
The inclusion of pozzolanic materials, such as fly ash or silica fume, can help mitigate ASR by consuming alkalis and reactive forms of silica, limiting their availability for the reaction. Pozzolanic materials also contribute to denser and more durable concrete, enhancing its resistance to ASR-induced expansion.
Proper concrete mix design is crucial in preventing ASR. The water-cement ratio should be optimized to minimize the availability of water for the reaction while maintaining adequate workability. The use of appropriate curing methods, such as moist curing, can help control moisture levels and prevent excessive drying, reducing the potential for ASR development.
5.3 Important points in concrete design and application stages:
Addressing ASR begins during the design and construction stages of concrete structures. Concrete mix designs should consider the reactivity of aggregates, alkali content, and environmental conditions to mitigate the risk of ASR. Thorough testing of aggregates for reactivity and assessing their potential contribution to ASR is essential.
During construction, proper curing practices should be implemented to maintain favorable moisture conditions in the concrete, promoting optimal hydration and reducing the risk of ASR. Moist curing, covering concrete surfaces with impermeable membranes, or using curing compounds can help maintain a moist environment, preventing excessive drying and minimizing ASR potential.
In conclusion, detecting ASR involves visual inspections, petrographic analysis, and non-destructive testing methods. Preventive measures encompass material selection, control of alkali content, utilization of pozzolanic materials, and proper concrete mix design. Additionally, attention to curing practices and environmental conditions during construction is crucial. By employing these detection and prevention strategies, the risk and impact of ASR can be effectively managed, ensuring the long-term durability and performance of concrete structures.
Case Studies and Solutions:
6.1 Real-life cases of alkali-silica reaction:
Examining real-life case studies of concrete structures affected by alkali-silica reaction (ASR) sheds light on the practical implications of this phenomenon and provides valuable insights into its impact and challenges faced. These case studies can encompass a range of structures, such as bridges, dams, parking structures, or buildings, which have experienced ASR-related issues.
In each case study, it is important to describe the specific symptoms and manifestations of ASR, such as the appearance of map-like cracks, spalling, or deformation. The extent of the damage and its implications on the functionality, safety, and durability of the structure should be highlighted, emphasizing the urgency of addressing ASR.
6.2 How the problem was solved or prevented:
Discussing the solutions and strategies employed to mitigate ASR in the case studies is crucial for understanding effective approaches to address this issue. It is essential to outline the preventive measures implemented during the design and construction stages to minimize the risk of ASR. This can include careful selection of aggregates with low reactivity, control of alkali content in cement and admixtures, and the use of pozzolanic materials to consume excess alkalis and reactive silica.
For existing structures affected by ASR, it is important to present the repairs and rehabilitation techniques employed to mitigate further damage and restore structural integrity. This can involve techniques such as crack injection, epoxy overlays, carbon fiber reinforcement, or even partial or complete replacement of affected elements.
Highlighting the lessons learned from these case studies is crucial in advancing our understanding of ASR and improving future practices. This can include insights into the importance of thorough material testing and selection, the significance of environmental monitoring, the effectiveness of preventive measures, and the value of early detection and timely intervention.
By examining these real-life cases and the solutions implemented, professionals in the field of concrete technology can gain valuable knowledge and guidance in managing ASR in their own projects. Sharing these experiences contributes to the collective understanding of ASR and helps in developing effective strategies for prevention, early detection, and appropriate remedial actions.
Ongoing Research and Advancements:
7.1 Research on ASR mechanisms and prevention techniques:
Continuous research efforts are dedicated to unraveling the complex mechanisms involved in alkali-silica reaction (ASR) and developing more effective techniques for prevention and mitigation. Scientists and engineers are conducting investigations to understand the molecular-level interactions between alkalis, silica, and other concrete constituents. By delving into the fundamental processes and factors influencing ASR, researchers aim to improve prediction models, develop new testing methods, and devise targeted prevention strategies.
Advanced analytical techniques, such as electron microscopy, spectroscopy, and X-ray diffraction, are employed to study the chemical and physical characteristics of ASR products. These studies provide valuable insights into the formation and properties of the alkali-silica gel, aiding in the identification of key factors that promote or inhibit ASR development. Additionally, computational modeling approaches are utilized to simulate the behavior of concrete under ASR conditions, enabling researchers to predict and analyze the long-term performance of structures.
7.2 Advancements in concrete technology:
In the quest to minimize the risk of ASR and enhance the durability of concrete structures, advancements in concrete technology are being pursued. Researchers are exploring alternative cementitious materials, such as geopolymers, which exhibit lower alkali content and reduced susceptibility to ASR. These materials offer promising potential for mitigating ASR-related issues in concrete construction.
Nanotechnology is also being applied to modify the reactivity of aggregates and control ASR. By incorporating nanoparticles or nano-sized additives into concrete, researchers aim to enhance the chemical stability of reactive silica, reducing its propensity to react with alkalis. This approach shows promise in developing ASR-resistant concrete mixes.
Furthermore, advancements in sensing technologies and monitoring systems are revolutionizing the detection and management of ASR. Integration of sensors within concrete structures enables real-time monitoring of ASR-related phenomena, such as moisture content, expansion, and internal stresses. Advanced imaging methods, such as ground-penetrating radar and infrared thermography, facilitate non-destructive evaluation of ASR-affected structures, aiding in early detection and targeted maintenance interventions.
Overall, ongoing research endeavors and advancements in concrete technology hold the promise of improved understanding, prevention, and management of ASR. By pushing the boundaries of knowledge and innovation, scientists and engineers are paving the way for more resilient and sustainable concrete structures.
International Standards and Guidelines:
8.1 Standards and guidelines for ASR prevention:
The prevention and management of alkali-silica reaction (ASR) are supported by various international organizations that have developed standards and guidelines to assist practitioners in mitigating the risks associated with ASR. These standards provide valuable recommendations and best practices for material selection, mix design, testing methods, and construction practices to minimize the occurrence and impact of ASR.
For instance, the American Concrete Institute (ACI) has published ACI 221R-96, "Guide for Use of Normal Weight and Heavyweight Aggregates in Concrete," which includes guidance on selecting aggregates to avoid potential ASR issues. The European Federation for Specialist Construction Chemicals and Concrete Systems (EFNARC) has also released guidelines that outline specific requirements and procedures to prevent ASR in concrete construction.
The International Union of Laboratories and Experts in Construction Materials, Systems, and Structures (RILEM) has contributed significantly to the field of ASR prevention through its technical committee on ASR. RILEM has published various reports and recommendations, including RILEM TC 106-ARP, "Accelerated Testing of Alkali-Reactivity of Concrete Aggregates," which focuses on accelerated laboratory testing methods for evaluating the potential for ASR in aggregates.
8.2 Harmonization of ASR-related standards:
The harmonization of ASR-related standards and guidelines is a significant step towards ensuring consistency and promoting the exchange of knowledge and best practices globally. Collaborative efforts among international organizations and research institutions are aimed at aligning approaches to ASR prevention and management, thereby facilitating the transfer of research findings and innovations across borders.
International conferences, symposiums, and workshops provide platforms for experts in the field to share their experiences and research outcomes, contributing to the harmonization of ASR-related practices. The exchange of information between professionals from different regions enhances the collective understanding of ASR and fosters the development of standardized approaches that can be universally applied.
Furthermore, initiatives led by organizations like the International Concrete Repair Institute (ICRI) and the International Federation for Structural Concrete (fib) promote the dissemination of ASR-related knowledge and encourage collaboration among professionals in the construction industry. These efforts aim to create a unified approach to ASR prevention and mitigation on a global scale.
By harmonizing ASR-related standards and guidelines, the international community can collectively address the challenges posed by ASR and work towards the development of more robust and sustainable concrete structures worldwide.
Future Directions and Challenges:
10.1 Addressing the complexity of ASR:
Despite significant progress in understanding ASR, there are still many aspects that require further exploration. ASR is a complex phenomenon influenced by numerous variables, including aggregate reactivity, cement chemistry, environmental factors, and construction practices. Future research should aim to unravel the intricate interactions among these factors and develop comprehensive models that can accurately predict ASR development and its long-term effects on concrete structures.
Advancements in analytical techniques, such as advanced microscopy, spectroscopy, and molecular modeling, can provide valuable insights into the molecular-level processes of ASR. By gaining a deeper understanding of the mechanisms and kinetics of ASR, researchers can develop more effective preventive measures and develop innovative materials that are resistant to the deleterious effects of ASR.
10.2 Sustainability considerations:
Sustainability has become a paramount concern in the construction industry, and addressing the impact of ASR on the environmental footprint of concrete structures is crucial. ASR-induced damage often necessitates repair or replacement, leading to additional resource consumption and energy expenditure. Minimizing the occurrence of ASR can contribute to the overall sustainability of concrete structures by reducing maintenance requirements, extending service life, and conserving natural resources.
To enhance the sustainability of concrete infrastructure, researchers and practitioners are exploring various avenues. This includes the development of low-alkali cements, the use of supplementary cementitious materials, and the optimization of mix designs to minimize the reactivity of aggregates. Additionally, incorporating life cycle assessment (LCA) methodologies into the decision-making process can help evaluate the environmental impact of ASR and guide the selection of sustainable construction practices.
Challenges in addressing ASR sustainability include balancing the trade-offs between mitigating ASR and ensuring other performance requirements of concrete structures, such as strength, durability, and workability. Finding a comprehensive and integrated approach that considers the environmental, economic, and social aspects of ASR prevention is vital for advancing sustainable concrete construction.
Furthermore, educating and raising awareness among engineers, architects, contractors, and other stakeholders about the importance of ASR prevention and sustainable practices is crucial. Collaborative efforts between researchers, industry professionals, and regulatory bodies are necessary to overcome the challenges and drive the adoption of sustainable strategies in ASR prevention and concrete construction.
In conclusion, addressing the complexity of ASR and incorporating sustainability considerations into ASR prevention are key directions for future research and practice. By advancing our understanding, developing innovative materials and preventive measures, and promoting sustainable construction practices, we can mitigate the impact of ASR on concrete structures and foster a more resilient and environmentally responsible built environment.
Conclusion:
In conclusion, the alkali-silica reaction (ASR) is a significant concern in the field of concrete technology, as it can lead to structural damage, reduced durability, increased maintenance costs, and safety hazards. Understanding the definition, chemical structure, and reaction mechanism of ASR provides the foundation for addressing this complex phenomenon.
The influence of various components in the concrete mixture, such as aggregates, cement composition, water-cement ratio, and alkali-containing admixtures, must be carefully considered to minimize the risk of ASR. Environmental factors, including temperature and moisture, also play a critical role in ASR development.
The consequences of ASR, such as swelling, cracking, and reduced concrete durability, have a detrimental impact on the structural integrity and longevity of concrete structures. The associated costs of repairing or replacing damaged elements further emphasize the importance of prevention.
Detection methods, such as visual inspections, petrographic analysis, and non-destructive testing techniques, enable the identification and assessment of ASR in concrete structures. Preventive measures, including material selection, control of alkali content, and proper concrete mix design, are essential to mitigate the risk of ASR.
Real-life case studies provide valuable insights into the impact of ASR and the solutions employed to prevent or address the issue. Sharing these experiences and lessons learned can inform best practices and guide future actions.
Ongoing research and advancements in concrete technology are focused on deepening our understanding of ASR mechanisms and developing more effective prevention techniques. By exploring alternative cementitious materials, nanotechnology, and advanced monitoring techniques, researchers aim to minimize the occurrence of ASR and enhance the durability of concrete structures.
International standards and guidelines provide valuable recommendations for ASR prevention, and efforts are underway to harmonize these standards globally. Collaboration among organizations and the exchange of knowledge facilitate consistent approaches to ASR prevention and foster innovation in the field.
Looking ahead, addressing the complexity of ASR and considering sustainability factors are important areas of focus. Advancing our understanding of ASR mechanisms, predictive modeling, and incorporating sustainability considerations in ASR prevention can contribute to the development of more resilient and environmentally responsible concrete structures.
In conclusion, by prioritizing ASR prevention, embracing advancements in concrete technology, adhering to international standards, and integrating sustainability principles, we can mitigate the detrimental effects of ASR and ensure the long-term performance and sustainability of concrete structures.
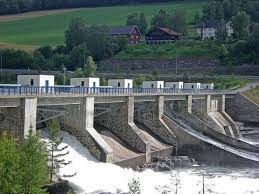
Comments